Novas Vs. Supernovas: What’s The Difference?
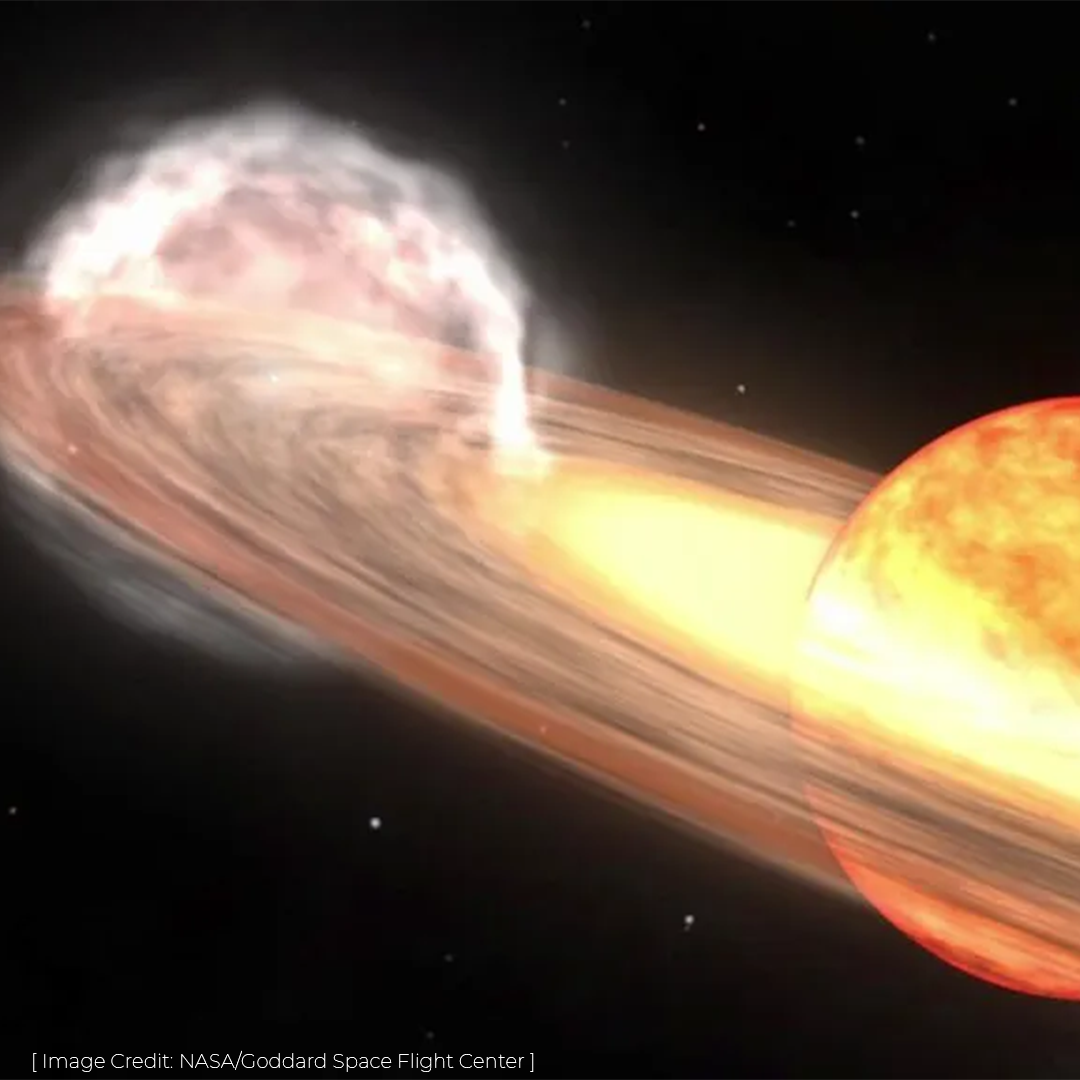
Header image: In this illustration, a white dwarf star and a red giant star orbit each other, resulting in a nova similar to the T Coronae Borealis nova that is seen every 80 years. Image credit: NASA/Goddard Space Flight Center
Written by Dr. Geza Gyuk, the Adler Planetarium’s Senior Director of Astronomy
Sometime in the summer of 2024, the otherwise unremarkable star T Coronae Borealis, also known as “the Blaze Star,” will flare dramatically. T Coronae Borealis is usually far below naked eye visibility, but for a brief few weeks, it will blaze brightly in our skies, peaking at more than a thousand times its usual brightness! From seemingly nothing, a new star—also known as a “nova”—will be born into our skies, only to fade away into obscurity.
How exactly do these novas form? What about supernovas? And what is the difference between the two? Let’s take a look at these two common types of stellar cataclysms.
Novas Vs. Supernovas
A supernova is much much brighter than a nova. While a nova will typically peak at around 100,000 times the luminosity of the Sun, a supernova is typically billions of times brighter than the Sun!
But the real difference between the two isn’t the brightness, it’s how the brightness is produced. In a nova, a star flares up and then returns to dormancy. In a supernova, the progenitor star is completely destroyed. How does this work?
What Is A Nova?
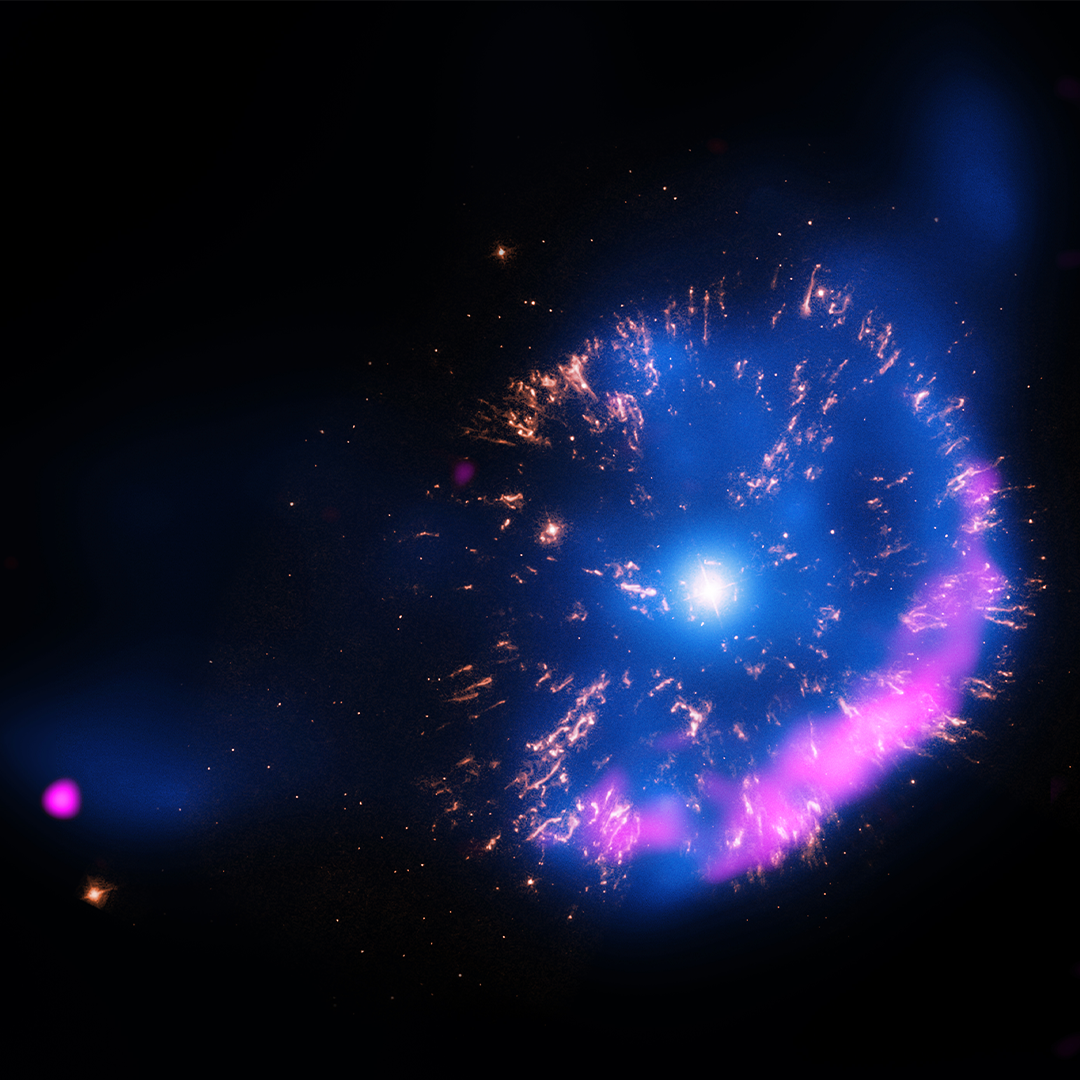
A nova actually starts as two stars. The first star in the binary is a white dwarf, the dead remnant core of a star like the Sun. A white dwarf has a star’s worth of matter packed into a volume about the size of the Earth. Its gravity is something fierce, not as strong as a black hole or even neutron star, but still a walloping 100,000–300,000 times that of the Earth! It also has a solid surface, unlike the Sun or most other stars which are composed of plasma.
The second, known as a companion star, is a regular star, made of hot incandescent hydrogen plasma. If the white dwarf orbits close enough to its companion star, its powerful gravity can actually steal some of its companion’s matter, pulling the plasma onto its own surface. There, the hydrogen piles up and is heated and squeezed by the white dwarf’s gravity. As the layer of stolen hydrogen gets ever thicker, denser, and hotter, it finally reaches a point where fusion reactions kick in and the hydrogen layer “burns” suddenly and explosively. In just minutes or hours, it may release more energy than the Sun produces in a thousand years! The remaining unburnt hydrogen is thrown out at speeds of more than 1,000 km/s.
As massive and impressive as a nova is, it is not powerful enough to destroy—or even seriously damage—the white dwarf or its companion. And so the process begins again with hydrogen accreted from the companion. For most novae, the time between outbursts will be many years. But for some novae, the time between reoccurrences is short enough that we have historical records of previous outbursts. T Coronae Borealis is one such “recurring nova”. It has a recurrence time of just under 80 years.
What Is A Supernova?
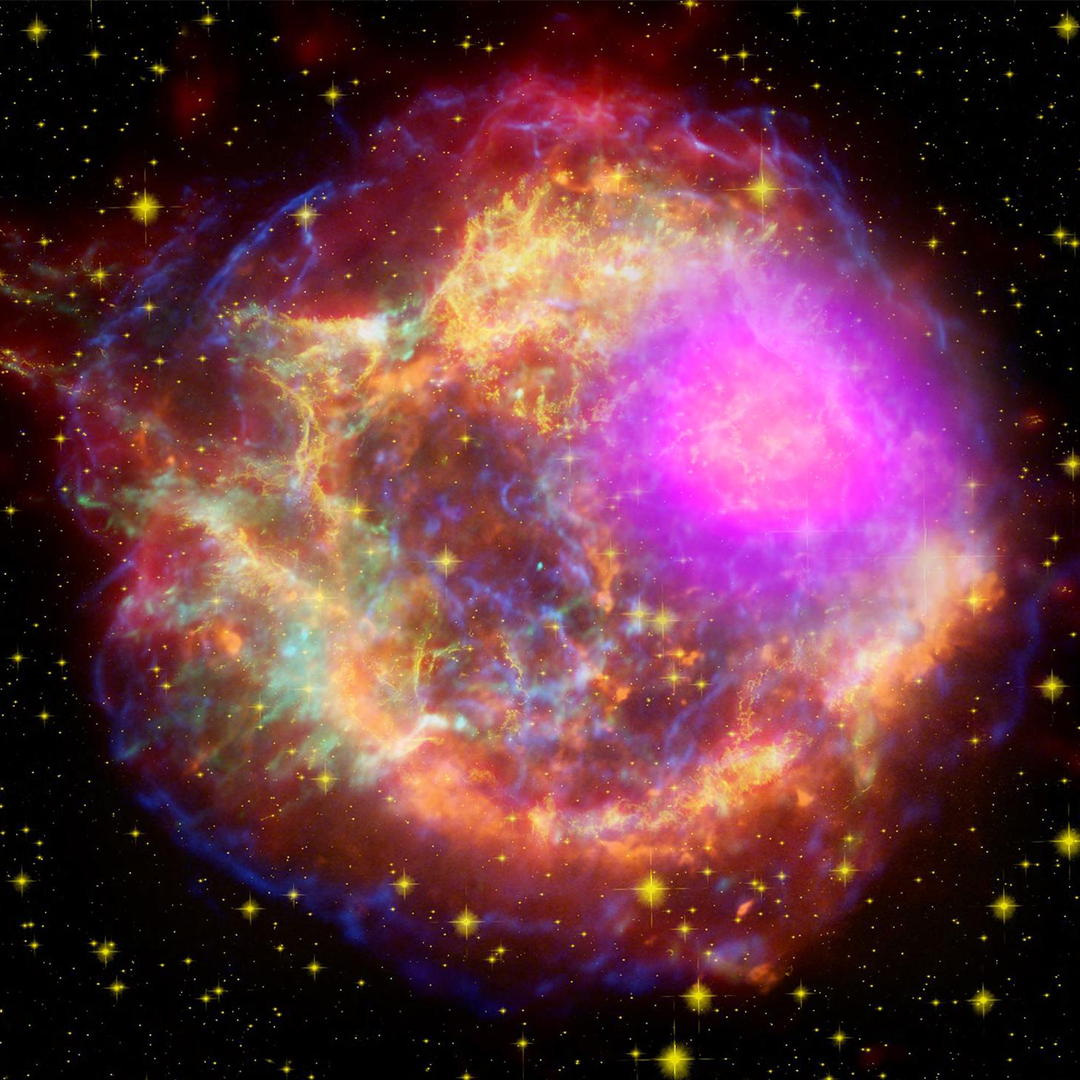
So if that’s a nova, then what powers a supernova? Well, there are two main kinds of supernovae, core collapse supernovae and thermal runaway supernovae. Sounds complicated, but hang on because the ride is wild but worth the trip!
A core collapse supernova is basically powered by gravity. That may not sound very impressive, but that’s because our experiences of gravity are based on small masses for small distances in small gravitational fields. If you drop a penny here on the Earth, it is a pretty forgettable event. But if you drop a watermelon from the top of a skyscraper, then we begin to see a hint of gravity’s power. Now, imagine you drop a watermelon from a skyscraper under a gravity a trillion times stronger than Earth’s…you’d expect a pretty powerful result when that watermelon hits the ground. In fact, the explosion would be equivalent to a 5 megaton bomb! The core of a star is much much more massive than a watermelon. And it is much bigger than a skyscraper. Put these things together and you have the recipe for something spectacular!
At the end of its life, the core of a massive star suddenly runs out of fuel. Without fuel for nuclear fusion, it can no longer support itself against its tremendous gravity—so it collapses. The most massive stars will collapse directly into a black hole. All that energy of the collapse simply gets swallowed up into the black hole and that’s that. But for stars of just the right mass, the core forms an absurdly dense and solid neutron star.
The neutron star serves as the “ground” off of which the falling mass can bounce, releasing its energy. The process of forming the neutron star actually releases the vast majority of the energy as a sudden fast burst of neutrinos which fly out of the doomed star. But the 1% that is left is enough to tear the star to bits, blowing the outer layers into space at speeds up to a few percent of the speed of light. That’s a supernova. Well, a type II, core collapse supernova, at any rate.
You’d think that nature would be satisfied with core collapse supernovae. But insanely, nature has figured out another way to make stars explode with absurd violence: thermal runaway supernovae.
Thermal runaway supernovae, also called Type Ia supernovae, are like a massively scaled up version of a regular nova. It starts with a white dwarf, which, in one way or another, increases in mass. A white dwarf is composed of carbon and oxygen atoms and the usual conditions inside a white dwarf cannot ignite nuclear fusion in these atoms. With no source of energy, the white dwarf just sits there, slowly cooling, radiating energy away as heat. But if the mass increases, then the pressure and temperature inside can grow due to the increased gravity and eventually it can reach a critical point. Deep within the core of the white dwarf, the sleeping dragon of fusion re-ignites.
Normally this wouldn’t be too terribly impressive. The fusion would produce a bit of heat, the heat would produce pressure, and the pressure would expand the star—lowering the temperature and the fusion rates would go down. The process would regulate itself. But the matter of a white dwarf is different.
In a white dwarf, the matter is degenerate—meaning an increase in temperature does NOT lead to increased pressure. So the white dwarf can’t expand and therefore, it can’t reduce the temperature. So more fusion leads to higher temperature, which leads to even more fusion, and so on. A runaway occurs and, in just a couple seconds, the entire white dwarf is consumed by a nuclear conflagration, raising temperatures to billions of degrees. The white dwarf is completely blown apart, with the debris racing outwards at velocities up to 6% of the speed of light.
The fundamental difference between a nova and a type Ia supernova is that the nuclear burning in a nova is hydrogen fusion and it happens in a layer on the surface. Only a tiny fraction of the mass of the star is burned, and so it lives to nova another day. In fact, it may be the case that over time, all nova recur and rebuild their mass, with each nova eruption leaving a deposit of ash on the white dwarf. Eventually many repeated novae may give birth to a supernova!
Learn More About T Coronae Borealis
With all the specifics of novas and supernovas now in your back pocket, learn about the upcoming nova that will brighten our skies this summer, T Coronae Borealis!
Join Experts At The Museum To Talk All Things Astronomy!
Do you have more questions about novas, supernovas, other stellar cataclysms, or anything else astronomy-related? Join us at the Adler Planetarium every Wednesday and Saturday for Astronomy Conversations! In Astronomy Conversations, visiting astronomers talk all things astronomy in an informal Q&A setting, paired with interactive space visualization experiences.
Learn more and see this month’s visiting experts!
Learn From Our Astronomy Educators!
Learn more about astronomy and the night sky in live episodes of Sky Observers Hangout! Learn how to observe upcoming cosmic happenings, enhance your astrophotography skills, and see celestial objects through a telescope virtually with our astronomy educators.
In their latest episode, our astronomy educators observe globular clusters through our Doane Observatory telescope in this cluster blockbuster! Globular clusters dazzle through telescopes with their tightly packed collections of thousands—or even millions—of stars. With some of these stars dating back to the early days of the universe, they give us insights into how stars form and evolve, and how galaxies collide and change.